Research areas: Geophysics, Mineral Physics
This project will investigate the coupled evolution of early crust formation and interior outgassing on Earth and Mars, which are strongly interlinked via the composition and chemistry in the planetary mantle, melting occurring in the deep mantle, and volatile speciation in the rising magma. Both the chemistry and the solubility of volatiles are affected by partial melting and melt composition. The focus during the first funding phase was on determining how volatiles and heat-producing elements partition into the crust and how chemical speciation occurs during planetary degassing, considering redox states that are representative of the early magmatic evolution of the Earth. Furthermore, in close cooperation with subproject C5, we confirmed the role of oxygen fugacity as one of the most important parameters affecting gas chemical speciation in the C-O-H system. We developed a geodynamical model of interior degassing that facilitates the simultaneous treatment of volcanic outgassing and gas speciation in rising magma bodies constrained by mantle chemistry and volatile bulk composition. Consequently, in the second phase of funding, we intend to strengthen our fruitful cooperation as we investigate the early evolution of rocky mantle and surface just after the latest stages of accretion of Mars and Earth, accounting for variations of the redox state of mantle and crustal melt over time. We will continue to develop an improved coupled model where the interior model will feed into the final gas speciation in the melt
- by tracing the fate of volatiles from mantle to surface, including partitioning of trace elements into the melt,
- by calculating the melt redox state from ultramafic to felsic compositions with respect to ambient temperature, dissolved volatiles and SiO2, and alkali content, and
- by modeling the amount of crust formation over time depending on the earliest thermal evolution of the planets after magma ocean solidification (C2/C4).
We will furthermore extend our speciation analyses to other gas phases such as halogens (Cl and F) and sulfur species and simulate time-dependent melt mixing scenarios, leading to diverse crust formation and outgassed volatile composition scenarios for early Earth and Mars under changing mantle redox states. For this we will investigate the effect of pressure on gas solubility by incorporating gas-melt equilibrium into the model. Moreover, we will invoke physical models of buoyant bubble rise, melt ascent and permeable outgassing to better constrain degassing rates. The cooperation with the climate model during Earth’s magma ocean solidification stage in C5 will be continued by modelling the changes in volatile fugacities in the magma ocean over time based on core formation models (C1).
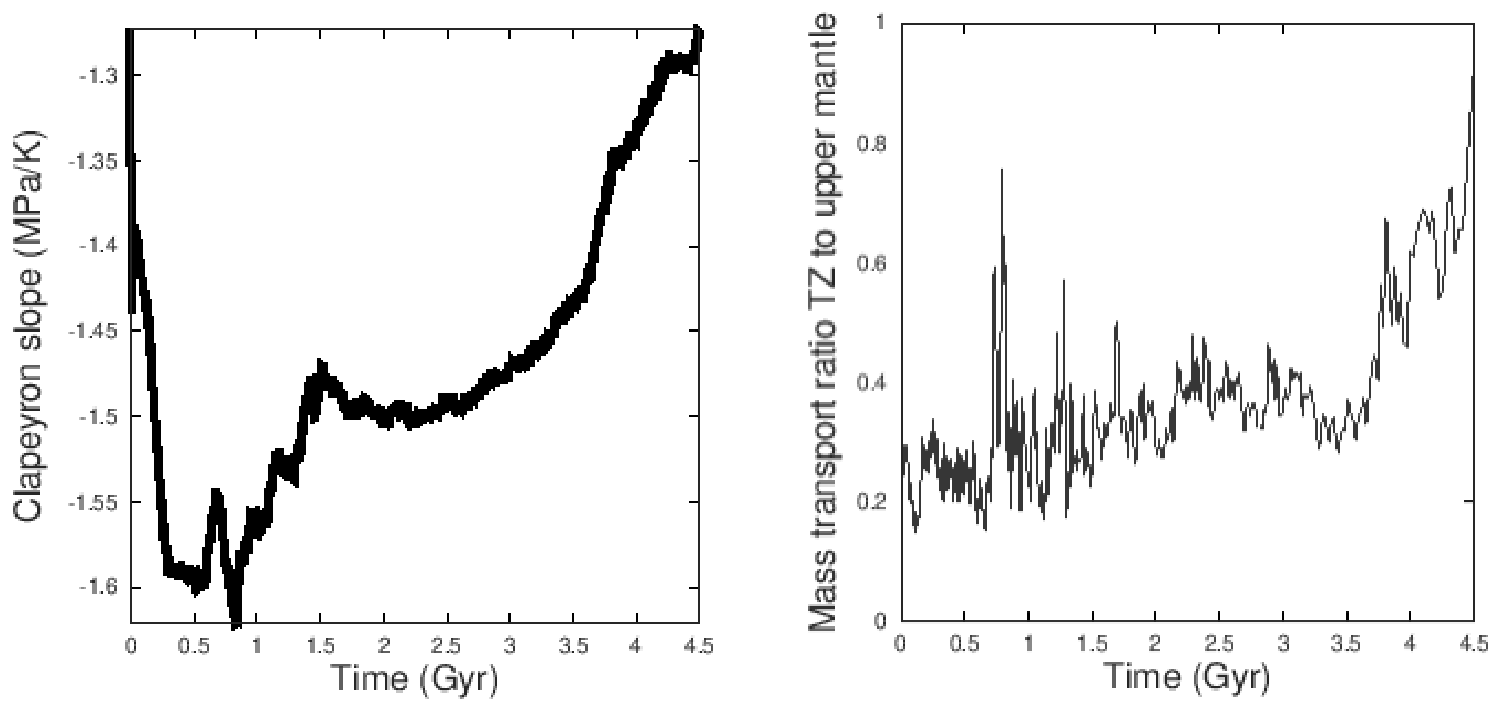
Figure: Model evolution of Clapeyron-Slope of the upper-lower mantle phase transition over time and resulting mixing efficiency (with 0=inhibited mass transport and 1=no effect of phase transition).
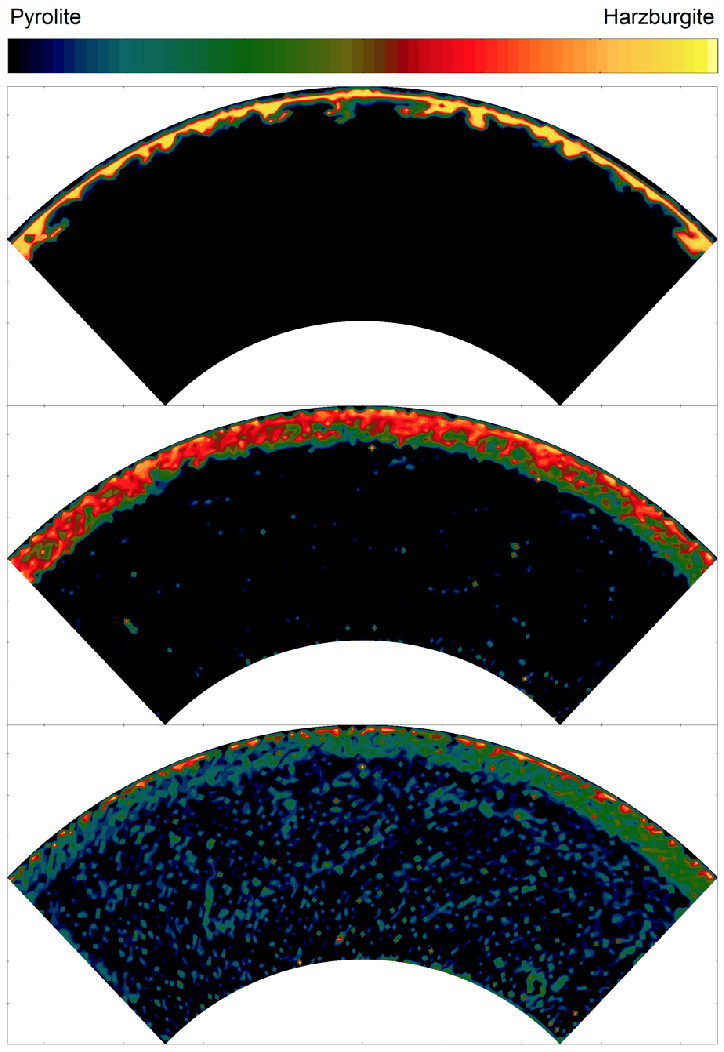
Figure: The chemical heterogeneities shown here are due to melt depletion from primordial, pyrolite composition (black) to depleted, harzburgite composition (yellow). To show the effect of the phase transition alone, here we neglect that depleted material is more buoyant than primordial mantle material. Melt depletion fields are shown (from top to bottom) for 50 Myr, 500 Myr and 2 Gyr after the last magma ocean solidification. Intermediate colours between black and yellow indicate mixed pyrolite-harzburgite material.